The theme of the research in the Jain lab hinges on the question: how can we use light to interface better with molecules and nanostructures? The goal is to use light in unique ways to: i) resolve important nanoscale or molecular processes that are not well understood, or ii) induce novel optoelectronic or photochemical behavior in matter. We are a diverse team with interest and expertise in spectroscopy, colloidal synthesis, nanofabrication, high-resolution electron microscopy, and plasmonics. The systems we investigate range from artificial photosynthetic systems to nanophotonic switches. Specific research areas are described below:

Catalysis
Super-Resolution Imaging of Heterogeneous Catalysts
Catalytic processes, despite their importance in the chemical industry as well as in solar-to-fuel conversion, remain poorly understood. This is primarily because of the involvement of surfaces that are often chemically complex and heterogeneous. In most cases, the identity of the active site is still in question. Our lab is using single-molecule super-resolution imaging techniques borrowed from the biophysics community, and high-resolution electron microscopy, to resolve individual active sites on a catalyst surface. By mapping the distribution, structural composition, and heterogeneity of active sites, we seek to enhance understanding of catalytic materials and processes. Particular focus is on catalysts for water-splitting and CO2 to methanol conversion.
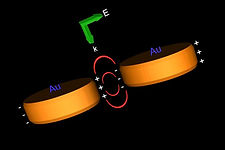
Plasmonics
Light-Matter Interactions
in the Near Field
The interaction of light with matter is primarily entailed by the excitation of electronic and vibrational modes by the electromagnetic field of light. The characteristic length scale of such excitations is typically on the molecular size scale (ca. 1 Å), whereas the characteristic length scale of the electromagnetic field can be defined for a plane wave by its wavelength (ca. 5000 Å for visible light). This disparity in length scales between a molecule and the electromagnetic field limits light-matter interactions to common dipole-type processes. By employing strong optical resonances of metal nanostructures to 'squeeze' electromagnetic fields down to the nanoscale (10 Å), our lab seeks to bridge the gap between light and molecular excitations and uncover novel photochemistry and photophysical behavior in quantum dots, metalloproteins, chiral molecules, photovoltaic, and photosynthetic systems. We are also interested in optoelectronic behavior of exotic material phases.
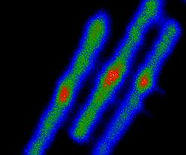
Single Nanocrystals
Imaging Phase Transitions in Single Nanocrystals
Phase transitions in solid-state materials often involve interesting dynamics. Since macroscopic solids are typically polycrystalline, such dynamics is smeared out in studies on bulk solids, due to ensemble averaging over different crystalline domains. By acquiring snapshots of a single nanocrystalline domain undergoing a phase transition, our lab is attempting to uncover the dynamic trajectory involved in the nucleation of a new phase. We are developing new optical and spectroscopic methods to acquire snapshots of model phase transitions and also using these techniques to learn new facts about fundamental phenomena such as crystal growth, impurity doping, and correlated electron systems.